The Agent
Anthrax is a serious disease that came into public prominence in 2001 during the bioterrorism attack in the United States. Anthrax is caused by a bacterium called Bacillus anthracis (B. anthracis). The name anthrax comes from the Greek word for coal and refers to the black skin lesions it produces. Descriptions of a disease affecting both animals and humans that appear to be anthrax have been found as early as Biblical times, and in fact anthrax has been suggested to have been the fifth plague described in the book of Exodus.
The anthrax bacterium was first described in 1823 and was the first bacterium ever shown to be the cause of a disease - in 1876, Robert Koch obtained a pure culture of B. anthracis and demonstrated that it caused disease by injecting it into animals. B. anthracis was also the first bacterium to be used, by Louis Pasteur in 1881, for making an attenuated vaccine.
B. anthracis is a large, rod-shaped bacterium that forms spores. These spores can survive in a dormant state in the environment, usually in soil, for many years, even decades. Once ingested, the spores are activated, and the bacteria begin to reproduce. Reproducing bacteria make three different proteins (protective antigen (PA), lethal factor (LF), and edema factor (EF)) that combine to form two toxins known as lethal toxin (composed of PA and LF) and edema toxin (composed of PA and EF). The toxins cause a fatal buildup of fluid around the lungs that can kill infected cells and produce disease and death in infected animals or humans.
Anthrax is primarily a disease of livestock that become infected by ingesting spores found in soil. Humans usually become infected with anthrax by handling products of infected animals such as leather or wool or by inhaling anthrax spores from infected animal products. They can also become infected by eating undercooked meat from infected animals. Anthrax is not known to be spread person-to-person. Cases of transmission of anthrax from infected animals to humans are relatively rare in the United States, with an average of about five cases per year.
However, in 2001, there were 22 cases of anthrax infection that were caused by deliberate spread through the United States Postal system. Letters containing anthrax killed five people and sickened 17 others. The attack caused a temporary disruption of mail service and forced the evacuation of several buildings including Senate offices and the Supreme Court. After a massive and difficult seven year investigation, the Federal Bureau of Investigation concluded this case after its leading and sole suspect, an Army microbiologist, committed suicide.
Three Main Forms of Human Anthrax
Cutaneous anthrax occurs when bacteria from infected animal products enter a break in the skin; black lesions occur at the site of infection. Other symptoms include fever and fatigue. This is the most common form of anthrax (greater than 95% of all cases). It can be controlled with antibiotics if it is treated before the infection spreads through the body; left untreated, it can be fatal in up to 20% of cases.
Gastrointestinal anthrax can result from the ingestion of contaminated meat that is eaten raw or undercooked. Symptoms include fever, nausea, vomiting, and abdominal pain. It can be fatal if not treated immediately.
Inhalation anthrax occurs when anthrax spores are inhaled. The spores travel to the lymph nodes near the lungs and produce toxins that cause severe breathing problems and shock. This form is the most dangerous because it is very difficult to treat and it is often fatal. Naturally occurring inhalation anthrax is very rare but, rather, occurs when anthrax spores are aerosolized. This was the type of anthrax infection that occurred during the bioterrorism attack of 2001; the letter attacks resulted in five deaths, in spite of intense antimicrobial therapy.
An additional form of anthrax has been identified in people who injected heroin, but no cases of injection anthrax have been reported in the United States.
The Problem
The bacterium that causes anthrax is considered a highly dangerous potential agent for use in bioterrorism. It is classified as a Category A agent – the highest risk type - by the Centers for Disease Control and Prevention (CDC). This means that if a bioterrorist attack were to happen, B. anthracis is considered to be one of the most likely agents to be used. The anthrax bacterium has been a central component to biological weapons research for over 60 years, in multiple countries including Japan, the former Soviet Union, and Great Britain.
The reasons that anthrax is so dangerous are that:
- it is highly toxic – the mortality rate is nearly 100% for the inhalation form, in the absence of treatment.
- its spores are easily disseminated through the air.
- the spores are extremely durable.
Although antibiotics exist that are effective against anthrax, the antibiotics must be administered shortly after exposure and before a person develops symptoms of anthrax disease. Because early symptoms are often confused with respiratory or gastrointestinal diseases, once the obvious symptoms occur it is usually too late to counteract the destructive effects of the anthrax toxins. There is also the serious concern that the anthrax bacterium could become resistant to currently used antibiotics. In fact, some strains are already resistant to certain classes of antibiotics.
There is an effective vaccine against the bacterium that causes anthrax, but its use is recommended only for adults aged 18 through 65 who have an occupational risk for anthrax exposure such as laboratory workers who handle B. anthracis, individuals who have direct contact with potentially infected livestock, and some military personnel. It is not recommended for the general public, and evaluation of its safety or effectiveness in children, pregnant women, the elderly, and people with weakened immune systems is incomplete. Therefore, most people would not be protected in the event of a bioterrorism attack.
While the vaccine has been licensed for some time for people before their possible exposure to anthrax due to occupational risk, it wasn't until November 2015 that the vaccine was approved for use in people after they have been exposed to anthrax. The new recommendation is for people of 18 through 65 years of age and calls for an accelerated schedule of shots. While the normal pre-exposure schedule of vaccination entails five shots over 18 months (with annual booster shots to maintain protection), people who have already been exposed would receive three shots of the vaccine over a four week period, along with a course of antibiotics.
So, although a vaccine exists, in the event of a large scale attack using anthrax as a bioweapon, there are concerns with the safety of the vaccine, the length of time it would take to acquire immunity to B. anthracis, and the availability of sufficient numbers of doses of the vaccine (or antibiotics) to protect the general population.
The Research
Much of the anthrax research being conducted within the department is focused on anthrax vaccines - both evaluation of the current licensed vaccine, as well as research into developing a new, improved vaccine. Other projects include identifying and analyzing the proteins that make up the anthrax bacterium, investigating mechanisms by which B. anthracis acquires the essential nutrient iron and overcomes a normal host defense against infection, and studying the problem of the resistance of B. anthracis to some antibiotics.
Evaluation of the licensed anthrax vaccine
The only licensed anthrax vaccine in the United States is called anthrax vaccine adsorbed (AVA, also known as BioThrax). This vaccine contains the anthrax protein protective antigen (PA), which elicits an antibody response from the host that provides immunity to anthrax.
The AVA vaccine has been shown to prevent anthrax disease. However, due to concerns about the safety of this vaccine for all population groups, the side effects of the vaccine, the number of doses and the time required to develop immunity, lot to lot variations in vaccine composition, and the possibility of insufficient supply in the event of a large scale anthrax attack, it is desirable to develop a new vaccine, or at least minimize the concerns of the existing one.
For over a decade, the Vaccine Research Center has been conducting studies and clinical trials to evaluate new ways of delivering the licensed AVA vaccine and to assess a diverse group of experimental anthrax vaccines. This work is being performed by Drs. Wendy Keitel, Hana El Sahly, Shital Patel, and their collaborators.
In one earlier study, Drs. Keitel and El Sahly took part in a CDC-sponsored phase 4 clinical trial of AVA with the goal of developing a simpler and better-tolerated way of giving the vaccine. Healthy volunteers were the given the standard number of AVA doses delivered under the skin or into the muscle, or reduced dose schedules into the muscle. The results indicated that the initial three or four doses of AVA given in the muscle provided immunological protection comparable to four standard injections under the skin, but with the benefit of significantly reducing the incidence of reactions at the site of injection.
This, and additional studies, demonstrated that it is possible to eliminate a dose, reducing the cost and increasing the number of doses available, and to change the administration route from the skin to the muscle, reducing the adverse effects at the injection site. This work has helped to guide the current recommendations for the pre-exposure anthrax vaccine regimen used with individuals having occupational risk for anthrax.
Drs. El Sahly and Patel have also been involved in studying the immunogenicity and safety of AVA for post-exposure treatment of anthrax in healthy adult volunteers. The goal of the trial was to evaluate the effect of reducing the amount of vaccine administered and reducing the number of doses, so that if an anthrax bioterrorism event should occur, the number of people receiving the vaccine could be maximized. In this study, participants received either the full dose of the vaccine or half the dose at days 0, 14, and 28, or they were vaccinated with the full dose at three different dosing schedules (days 0 and 14, days 0 and 28, or days 0, 14, and 28). They found that the half dose regimen did not induce antibody production as well as the full dose, and that the subjects who received the vaccine at day 28 had higher antibody levels that persisted longer than subjects who did not receive the vaccine at day 28.
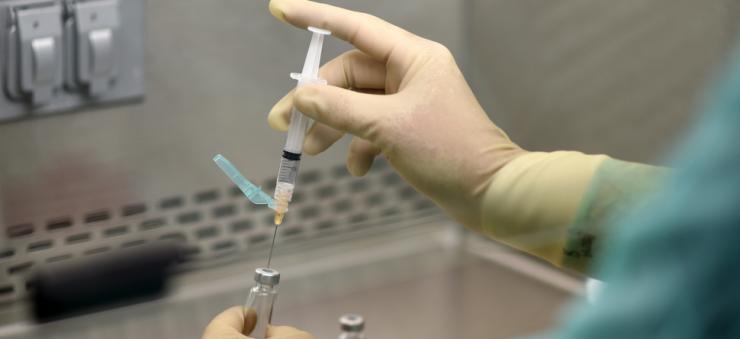
Development of new anthrax vaccines
Although minimizing side effects and reducing the number of doses needed of the current vaccine is beneficial, the ultimate goal is to develop a safe, more effective vaccine that would provide immunity to anthrax within a shorter period of time. To rationally develop a new anthrax vaccine, scientists need to understand how the anthrax vaccine works in humans to elicit an antibody response and provide protection.
For this purpose, Dr. Keitel and colleagues conducted studies to analyze in detail the interactions between PA, the primary immunogenic component of the current vaccine, as well as proposed next-generation anthrax vaccines, and the antibodies produced in humans. The results of this work can guide researchers in the design of more effective anthrax vaccines.
One candidate for a new anthrax vaccine that has been investigated is based on a purified recombinant PA protein (rPA102). The safety and immunogenicity of the rPA120 vaccine at different dosage levels were evaluated in a phase I clinical trial. The conclusions of this study were that the rPA120 vaccine did not cause serious side effects, and that the vaccine was effective in producing an anti-PA antibody response that increased with increasing vaccine dose levels and number of doses. Neutralization activity at the highest rPA120 dosage was similar to that seen in subjects receiving AVA injections.
Another candidate for an anthrax vaccine is composed of two segments of DNA that express PA and lethal factor (LF), two of the three components of anthrax toxins. In a VTEU-supported study, Drs. Keitel and El Sahly and colleagues, evaluated the safety of the DNA-based vaccine in healthy human volunteers and tested whether it could stimulate antibody responses that are associated with protection against infection. They found that the vaccine was generally well tolerated and that a greater percentage of subjects developed antibodies to PA or LF in the groups that received the higher doses of the vaccine.
The researchers further tested the DNA-based anthrax vaccine in nonhuman primates to evaluate immunogenicity and to determine if the vaccine could provide protection against challenge with a lethal dose of B. anthracis spores. Low levels of antibody were detected in the monkeys after vaccination. Importantly, 75% of the animals survived the lethal challenge. They concluded that the vaccine provided immunity at doses that are generally well tolerated by humans.
Dr. Joseph Petrosino and colleagues have been using an approach called functional genomics to identify and study B. anthracis proteins involved in conferring virulence to the bacterium. Two independent virulence plasmids (pXO1 and pXO2) distinguish B. anthracis from other Bacillus species. These plasmids encode 224 genes, including the three anthrax toxin subunits (PA, LF, and EF). The researchers used a novel technique that allowed them to analyze this large group of genes. The virulence plasmid genes were cloned, and their proteins were expressed and purified and used in screens to identify which were immunoreactive with serum from three different anthrax infection animal models, as well as from AVA-vaccinated humans. The anthrax proteins identified in such a screen would be possible candidates for the development of a new generation of anthrax vaccines.
Their study identified ten proteins that were detected by antibodies from all three anthrax animal models (rabbits, mice, and rhesus macaque monkeys); eight of these proteins had not previously been shown to be immunoreactive. When they tested serum obtained from humans who had received the AVA vaccine with the purified proteins, they detected an expected response to PA, but no response with any of the other proteins, suggesting that antibodies elicited by the current vaccine do not react with the proteins they identified using the animal models.
These results indicate that a multi-component subunit vaccine, containing PA and another protein, rather than PA alone, may provide greater protection, because antibodies would be raised to additional targets. Further work is needed to reveal which of the candidates identified in this study could be combined with PA to create an improved vaccine against anthrax.
An additional candidate that could potentially serve as an antigen for an anthrax vaccine is a recombinant heme transporter. Dr. Anthony Maresso has been investigating iron acquisition by B. anthracis. Iron is an essential nutrient used by almost all organisms. Bacterial pathogens must acquire iron in order to grow inside mammalian hosts. The host, however, limits the availability of free iron, thereby providing an effective defense strategy against infection. In response, bacteria have evolved clever ways to subvert host sequestration of iron. Dr. Maresso has uncovered five secreted proteins produced by B. anthracis which specifically bind and transport the body’s prominent iron-carrier molecule, heme. These proteins contain a conserved heme-binding domain referred to as the near-iron transporter (NEAT).
Dr. Maresso, along with Dr. Keitel and others, conducted a study to determine if recombinant NEAT proteins can protect against anthrax disease. They found that vaccination with the NEAT proteins protected mice from B. anthracis infection, and they characterized the immune response elicited by the vaccine in a mouse model of B. anthracis infection. They further obtained the crystal structure of one of the NEAT proteins and identified important functional parts of the protein. These results indicate that NEAT proteins should be considered in the development of an improved vaccine against anthrax and this work can be used to guide this development.
In a separate line of research, Dr. Maresso has identified and studied a group of small molecule inhibitors which specifically target and inactivate enzymes in the pathway of iron acquisition in pathogens like B. anthracis. An understanding of the mechanisms of iron uptake in B. anthracis will allow for the development of therapeutic agents to combat infections by related gram-positive bacteria.
Antibiotic resistance
In addition to the administration of the anthrax vaccine for someone who has been exposed to anthrax, treatment for anthrax infections includes use of the antibiotics ciprofloxacin, doxycycline, and penicillin G. The problem is that some strains of the anthrax bacterium are naturally resistant to penicillin, so that penicillin is ineffective in treating patients with these particular strains. Resistance to penicillin is often due to enzymes made by bacteria that are called β-lactamases (these enzymes basically break apart penicillin). One strain of B. anthracis makes two such enzymes (called Bla1 and Bla2). Dr. Timothy Palzkill and his group are studying these enzymes to learn in detail how they allow some strains of B. anthracis to resist antibiotics. Their goal is to develop compounds that can inhibit the action of the β-lactamase enzymes, so that they are no longer effective in blocking the action of antibiotics.
For More Information
Glossary
Learn more about some of the technical terms found on our glossary of terms page.