Quantitative Real-Time Imaging of Glutathione Dynamics
We developed a glutathione (GSH) probe—RealThiol—that allows real-time monitoring of GSH dynamics in living cells (Nature Communications 2017).
Impact of Our GSH Probes
Global distribution of GSH probes developed by our group. The GSH probes, including RealThiol (RT) and Mito-RealThiol (Mito-RT) probes, have been distributed to over 50 institutions worldwide through either material transfer agreements (MTAs) or Kerafast.com. The map is updated on Dec 27, 2018.
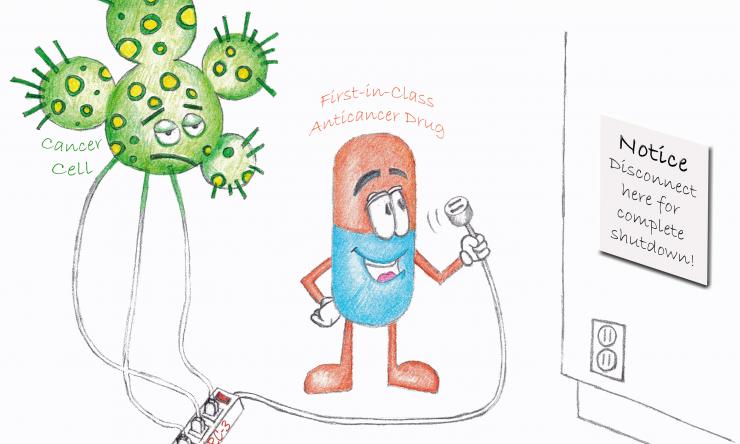
Steroid Receptor Coactivator 3 (SRC-3) Small Molecule Inhibitors
We developed a small molecule inhibitor that can inhibitor SRC-3 and impact many cancer signaling pathways simultaneously (PNAS 2016).
About the Lab
We are a highly interdisciplinary group and interested in developing tools for biological and biomedical studies. Currently, our research is mainly focused in three areas:
- Developing novel fluorescent probes to quantitatively monitor real-time glutathione dynamics in living cells
- Developing small molecule inhibitor against coactivators to treat breast cancer
- Developing antibody drug conjugates as novel cancer therapies